期刊全称 | Bioreactor Systems for Tissue Engineering | 影响因子2023 | Cornelia Kasper,Martijn Griensven,Ralf Pörtner | 视频video | | 发行地址 | Covers trends in modern biotechnology.All aspects of this interdisciplinary technology, where knowledge, methods and expertise are required from chemistry, biochemistry, microbiology, genetics, chemic | 学科分类 | Advances in Biochemical Engineering/Biotechnology | 图书封面 | 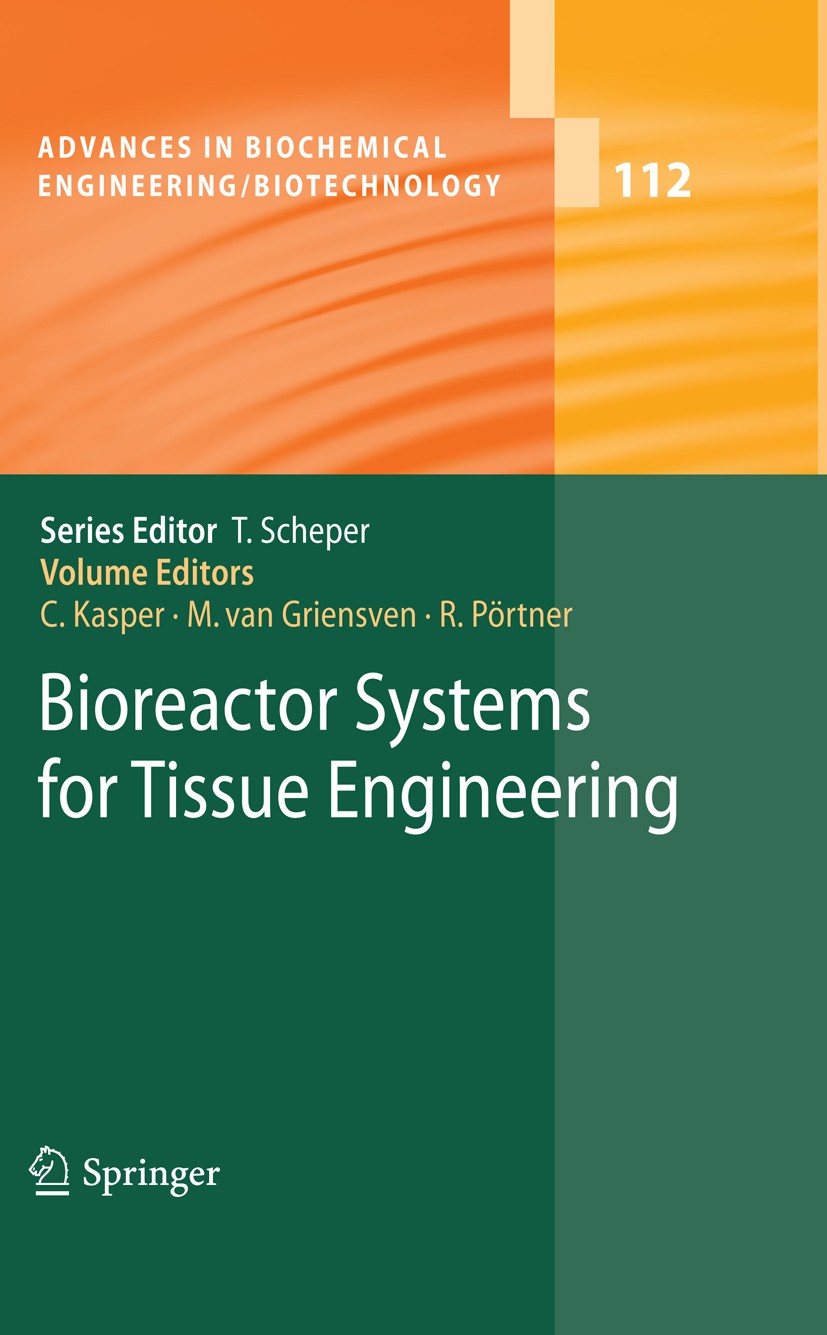 | 影响因子 | The editors of this special volume would first like to thank all authors for their excellent contributions. We would also like to thank Prof. Dr. Thomas Scheper, Dr. Marion Hertel and Ulrike Kreusel for providing the opportunity to compose this volume and Springer for organizational and technical support. Tissue engineering represents one of the major emerging fields in modern b- technology; it combines different subjects ranging from biological and material sciences to engineering and clinical disciplines. The aim of tissue engineering is the development of therapeutic approaches to substitute diseased organs or tissues or improve their function. Therefore, three dimensional biocompatible materials are seeded with cells and cultivated in suitable systems to generate functional tissues. Many different aspects play a role in the formation of 3D tissue structures. In the first place the source of the used cells is of the utmost importance. To prevent tissue rejection or immune response, preferentially autologous cells are now used. In particular, stem cells from different sources are gaining exceptional importance as they can be differentiated into different tissues by using special | Pindex | Book 2009 |
1 |
Front Matter |
|
|
Abstract
|
2 |
,Bioreactors in Tissue Engineering: Scientific Challenges and Clinical Perspectives, |
D. Wendt,S. A. Riboldi,M. Cioffi,I. Martin |
|
Abstract
In this Chapter we discuss the role of bioreactors in the translational paradigm of Tissue Engineering approaches from basic research to streamlined tissue manufacturing. In particular, we will highlight their functions as: (1) ., making up for limitations of conventional manual and static techniques, enabling automation and allowing physical conditioning of the developing tissues; (2) ., enabling us to recapitulate specific aspects of the actual in vivo milieu and, when properly integrated with . techniques, to address challenging scientific questions; (3) ., implementing bioprocesses so as to support safe, standardized, scaleable, traceable and possibly cost-effective production of grafts for clinical use. We will provide evidences that fundamental knowledge gained through the use of well-defined and controlled bio-reactor systems at the research level will be essential to define, optimize, and moreover, . the key processes required for efficient manufacturing models.
|
3 |
,Bioreactor Technology in Cardiovascular Tissue Engineering, |
H. Mertsching,J. Hansmann |
|
Abstract
Cardiovascular tissue engineering is a fast evolving field of biomedical science and technology to manufacture viable blood vessels, heart valves, myocar-dial substitutes and vascularised complex tissues. In consideration of the specific role of the haemodynamics of human circulation, bioreactors are a fundamental of this field. The development of perfusion bioreactor technology is a consequence of successes in extracorporeal circulation techniques, to provide an in vitro environment mimicking in vivo conditions. The bioreactor system should enable an automatic hydrodynamic regime control. Furthermore, the systematic studies regarding the cellular responses to various mechanical and biochemical cues guarantee the viability, bio-monitoring, testing, storage and transportation of the growing tissue..The basic principles of a bioreactor used for cardiovascular tissue engineering are summarised in this chapter.
|
4 |
,Bioreactors for Guiding Muscle Tissue Growth and Development, |
R. G. Dennis,B. Smith,A. Philp,K. Donnelly,K. Baar |
|
Abstract
Muscle tissue bioreactors are devices which are employed to guide and monitor the development of engineered muscle tissue. These devices have a modern history that can be traced back more than a century, because the key elements of muscle tissue bioreactors have been studied for a very long time. These include barrier isolation and culture of cells, tissues and organs after isolation from a host organism; the provision of various stimuli intended to promote growth and maintain the muscle, such as electrical and mechanical stimulation; and the provision of a perfusate such as culture media or blood derived substances. An accurate appraisal of our current progress in the development of muscle bioreactors can only be made in the context of the history of this endeavor. Modern efforts tend to focus more upon the use of computer control and the application of mechanical strain as a stimulus, as well as substrate surface modifications to induce cellular organization at the early stages of culture of isolated muscle cells.
|
5 |
,Bioreactors for Connective Tissue Engineering: Design and Monitoring Innovations, |
A. J. El Haj,K. Hampson,G. Gogniat |
|
Abstract
The challenges for the tissue engineering of connective tissue lie in creating off-the-shelf tissue constructs which are capable of providing organs for transplantation. These strategies aim to grow a complex tissue with the appropri ate mechanical integrity necessary for functional load bearing. Monolayer culture systems lack correlation with the in vivo environment and the naturally occur ring cell phenotypes. Part of the development of more recent models is to create growth environments or bioreactors which enable three-dimensional culture. Evidence suggests that in order to grow functional load-bearing tissues in a bioreactor, the cells must experience mechanical loading stimuli similar to that experienced in vivo which sets out the requirements for mechanical loading bioreactors. An essential part of developing new bioreactors for tissue growth is identifying ways of routinely and continuously measuring neo-tissue formation and in order to fully identify the successful generation of a tissue implant, the appropriate on-line monitoring must be developed. New technologies are being developed to advance our efforts to grow tissue ex vivo. The bioreactor is a critical part of thes
|
6 |
,Mechanical Strain Using 2D and 3D Bioreactors Induces Osteogenesis: Implications for Bone Tissue En |
M. van Griensven,S. Diederichs,S. Roeker,S. Boehm,A. Peterbauer,S. Wolbank,D. Riechers,F. Stahl,C. K |
|
Abstract
Fracture healing is a complicated process involving many growth factors, cells, and physical forces. In cases, where natural healing is not able, efforts have to be undertaken to improve healing. For this purpose, tissue engineering may be an option. In order to stimulate cells to form a bone tissue several factors are needed: cells, scaffold, and growth factors. Stem cells derived from bone marrow or adipose tissues are the most useful in this regard. The differentiation of the cells can be accelerated using mechanical stimulation. The first part of this chapter describes the influence of longitudinal strain application. The second part uses a sophisticated approach with stem cells on a newly developed biomaterial (Sponceram) in a rotating bed bioreactor with the administration of bone morphogenetic protein-2. It is shown that such an approach is able to produce bone tissue constructs. This may lead to production of larger constructs that can be used in clinical applications.
|
7 |
,Bioreactors for Tissue Engineering of Cartilage, |
S. Concaro,F. Gustavson,P. Gatenholm |
|
Abstract
The cartilage regenerative medicine field has evolved during the last decades. The first-generation technology, autologous chondrocyte transplantation (ACT) involved the transplantation of in vitro expanded chondrocytes to cartilage defects. The second generation involves the seeding of chondrocytes in a three-dimensional scaffold. The technique has several potential advantages such as the ability of arthroscopic implantation, in vitro pre-differentiation of cells and implant stability among others (Brittberg M, Lindahl A, Nilsson A, Ohlsson C, Isaksson O, Peterson L, N Engl J Med 331(14):889–895, 1994; Henderson I, Francisco R, Oakes B, Cameron J, Knee 12(3):209–216, 2005; Peterson L, Minas T, Brittberg M, Nilsson A, Sjogren-Jansson E, Lindahl A, Clin Orthop (374):212–234, 2000; Nagel-Heyer S, Goepfert C, Feyerabend F, Petersen JP, Adamietz P, Meenen NM, et al. Bioprocess Biosyst Eng 27(4):273–280, 2005; Portner R, Nagel-Heyer S, Goepfert C, Adamietz P, Meenen NM, J Biosci Bioeng 100(3):235–245, 2005; Nagel-Heyer S, Goepfert C, Adamietz P, Meenen NM, Portner R, J Biotechnol 121(4):486–497, 2006; Heyland J, Wiegandt K, Goepfert C, Nagel-Heyer S, Ilinich E, Schumacher U, et al. Biot
|
8 |
,Technical Strategies to Improve Tissue Engineering of Cartilage-Carrier-Constructs, |
R. Pörtner,C. Goepfert,K. Wiegandt,R. Janssen,E. Ilinich,H. Paetzold,E. Eisenbarth,M. Morlock |
|
Abstract
Technical aspects play an important role in tissue engineering. Especially an improved design of bioreactors is crucial for cultivation of artificial three-dimensional tissues in vitro. Here formation of cartilage-carrier-constructs is used to demonstrate that the quality of the tissue can be significantly improved by using optimized culture conditions (oxygen concentration, growth factor combination) as well as special bioreactor techniques to induce fluid-dynamic, hydrostatic or mechanical load during generation of cartilage.
|
9 |
,Application of Disposable Bag Bioreactors in Tissue Engineering and for the Production of Therapeut |
R. Eibl,D. Eibl |
|
Abstract
In order to increase process efficiency, many pharmaceutical and biotechnology companies have introduced disposable bag technology over the last 10 years. Because this technology also greatly reduces the risk of cross-contamination, disposable bags are preferred in applications in which an absolute or improved process safety is a necessity, namely the production of functional tissue for implantation (tissue engineering), the production of human cells for the treatment of cancer and immune system diseases (cellular therapy), the production of viruses for gene therapies, the production of therapeutic proteins, and veterinary as well as human vaccines..Bioreactors with a pre-sterile cultivation bag made of plastic material are currently used in both development and manufacturing processes primarily operating with animal and human cells at small- and middle-volume scale. Because of their scalability, hydrodynamic expertise and the convincing results of oxygen transport efficiency studies, wave-mixed bioreactors are the most used, together with stirred bag bioreactors and static bags, which have the longest tradition..Starting with a general overview of disposable bag bioreactors and th
|
10 |
,Methodology for Optimal In Vitro Cell Expansion in Tissue Engineering, |
J.M. Melero-Martin,S. Santhalingam,M. Al-Rubeai |
|
Abstract
Expansion of the cell population in vitro has become an essential step in the process of tissue engineering and also the systematic optimization of culture conditions is now a fundamental problem that needs to be addressed. Herein, we provide a rational methodology for searching culture conditions that optimize the acquisition of large quantities of cells following a sequential expansion process. In particular, the analysis of both seeding density and passage length was consid ered crucial, and their correct selection should be taken as a requisite to establish culture conditions for monolayer systems. This methodology also introduces addi tional considerations concerning the running cost of the expansion process. The selection of culture conditions will be a compromise between optimal cell expan sion and acceptable running cost. This compromise will normally translate into an increase of passage length further away from the optimal value dictated by the growth kinetic of the cells. Finally, the importance of incorporating functional assays to validate the phenotypical and functional characteristics of the expanded cells has been highlighted. The optimization approach presented wil
|
11 |
,Bioreactor Studies and Computational Fluid Dynamics, |
H. Singh,D. W. Hutmacher |
|
Abstract
The hydrodynamic environment “created” by bioreactors for the culture of a tissue engineered construct (TEC) is known to influence cell migration, proliferation and extra cellular matrix production. However, tissue engineers have looked at bioreactors as black boxes within which TECs are cultured mainly by trial and error, as the complex relationship between the hydrodynamic environment and tissue properties remains elusive, yet is critical to the production of clinically useful tissues. It is well known in the chemical and biotechnology field that a more detailed description of fluid mechanics and nutrient transport within process equipment can be achieved via the use of computational fluid dynamics (CFD) technology. Hence, the coupling of experimental methods and computational simulations forms a synergistic relationship that can potentially yield greater and yet, more cohesive data sets for bioreactor studies. This review aims at discussing the rationale of using CFD in bioreactor studies related to tissue engineering, as fluid flow processes and phenomena have direct implications on cellular response such as migration and/or proliferation. We conclude that CFD should be seen by
|
12 |
,Fluid Dynamics in Bioreactor Design: Considerations for the Theoretical and Practical Approach, |
B. Weyand,M. Israelowitz,H. P. von Schroeder,P. M. Vogt |
|
Abstract
The following chapter summarizes principles of fluid dynamics in bioreactor design with a focus on mammalian cell-culture systems.
|
13 |
Back Matter |
|
|
Abstract
|
|
|